atomic clocks comparisons
We are quite proud that our time to digital converters resolve so accurately that they are suitable for comparing atomic clocks. This applies in particular to modern measurement methods, such as two-way satellite time and frequency transfer.
All common clocks work according to a similar principle: They use a periodically occurring event, which is counted and their frequency is assigned to a second. Only with the help of atomic clocks time has become the physical quantity that can be measured most precisely. The time pulse of these clocks is derived from the characteristic frequency of radiative transitions of the electrons of free atoms, and their time display is continuously compared with and adapted to the clock. Worldwide, more than 400 atomic clocks are operated in 80 time institutes, which are regularly compared to each other. Based on this comparison a kind of "averaged" time scale is calculated, which is called the International Atomic Time (TAI) and is used worldwide. The TAI is also the basis for the Coordinated Universal Time (UTC).
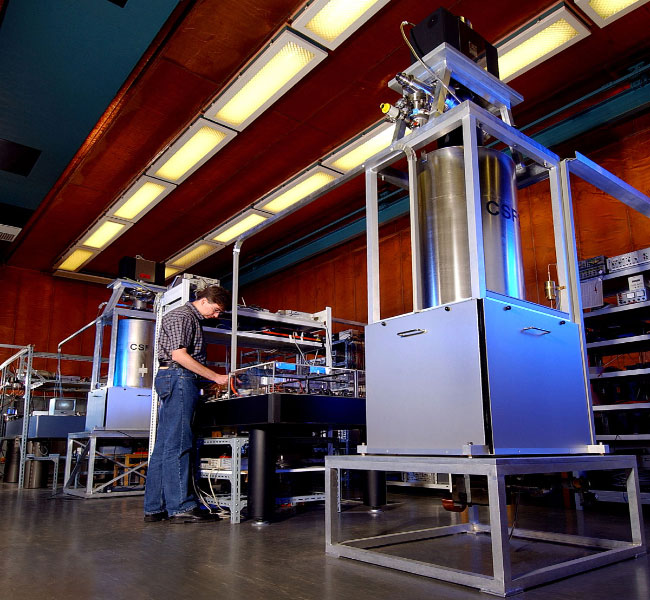
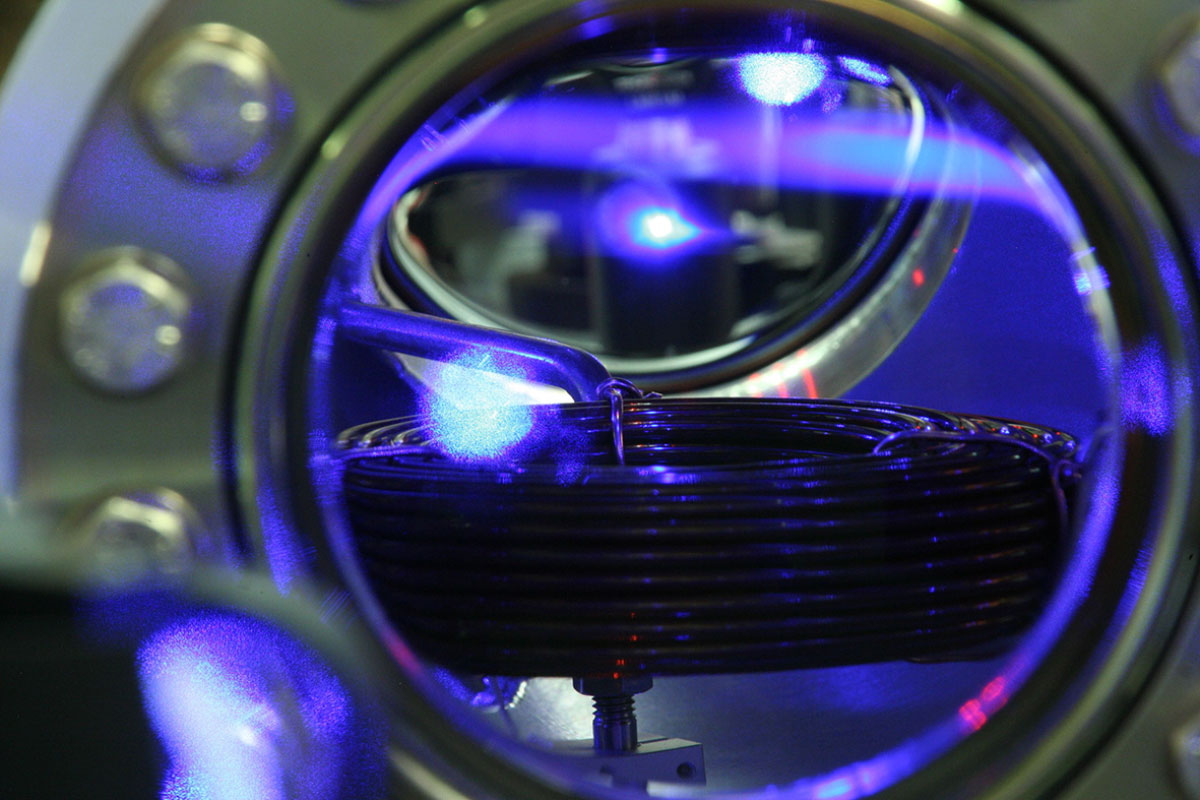
How are atomic clocks synchronized?
The availability of GPS time comparisons was a significant step to compare distant atomic clocks. The above-mentioned satellite network provides besides the position data for navigation systems also the exact time signals and is therefore basically suitable for the time dissemination and for the comparison of time scales. For this purpose, a GPS receiver in each time institute is synchronized with the UTC of the institute and the time difference between this time indication and that of the satellite GPS is calculated. In this process, each satellite transmits the system time as a reference together with the value from the time scale of the individual onboard atomic clock and the current position data. Thus, the relationship between the individual satellite clock and the system time can be used in a time comparison.
Using this method, if several institutes determine time differences from observations of all satellites during one day and exchange their results, this allows the calculation of the average time difference. However, this method has systematic disadvantages, especially because the satellites emit only a weak signal. Consequently, the data might be manipulated by spoofing or spamming.
Therefore, the method of two-way satellite time and frequency transfer (TWSTFT) has become more established today. In this approach, two time institutes simultaneously send timestamps at an agreed time to each other via a geostationary telecommunication satellite. These timestamps provide the exact send time according to the self-measured atomic time. At the time of sending, a TDC is started at each of the two transmitters and automatically stopped as soon as the signal of the other institute is received. By comparing the transmission times according to the atomic clock of one institute with the transmission times according to the TDC of the other institute, the frequency or time difference of the two distant atomic clocks can be determined. In this process, the measurement is independent of the position of the two institutes and the satellite, since the delays acting on the signals usually affect both signals equally. Consequently, they neutralize each other in the computational comparison.

Satellite links are not adequate for the comparison of optical clocks, because the achieved better precision of the time signal is lost in the noise of the transmission. This sparked the idea of coupling several optical clocks via laser beams transmitted by fiber-optic technology. The approach has already succeeded in several experiments, including one in Boulder in 2021 with three atomic clocks located about 1.5 kilometers apart, which could be precisely synchronized with each other over a period of eight months. The measured clock rate differences were only noticeable after the seventeenth decimal place, which corresponded approximately to the accuracy of each individual atomic clock. The long-term goal is to build a global network of optical atomic clocks.
What is the benefit of the extraordinary accuracy of atomic clocks?
Even if most people think that a time measurement accurate to the second is sufficient for them in everyday life, we all benefit indirectly from the existence and the global synchronization of atomic clocks. This is not only because they set the pace for radio-controlled clocks and station clocks. Exact time measurement plays a major role in research in particular, but also in world trade and navigation, for example. There are many examples of applications, some of which we would like to mention here.
- Probably the best-known application for atomic clocks is time transmission via time signal radio transmitters. Many governments operate transmitters for timekeeping, which are available for a wide variety of instruments and have a transit delay of about 1 ms per 300 km from the radio transmitter. Conventional radio clocks also synchronize automatically with the atomic clocks used for this purpose, but they rely exclusively on the amplitude-modulated time signals and use narrowband receivers (with 10 Hz bandwidth) with very small ferrite loop antennas. Because of their low-cost signal processing, they can determine the beginning of seconds only with a practical inaccuracy of ± 0.1 seconds. Immediately after a successful synchronization they are most accurate and become increasingly inaccurate from then on until the next synchronization.
- A particularly widespread application for atomic clocks is, of course, satellite navigation, which is based on time-of-flight measurements of signals transmitted by satellites. All the individual satellites of the American GPS, the European Galileo, the Russian Glonass, and the Chinese Beidou satellites are equipped with an atomic clock. From the travel times of the signals of several satellites, the position can be determined with receiving devices. The accuracy of the time measurement plays a central role. Even a deviation of only a millionth of a second will cause the determined position of the receiver to be wrong by several hundred meters. The better the atomic clocks of the satellites are synchronized, the more precise positions can be determined using satellite navigation.
- In radio astronomy (VLBI), atomic clocks are used, for example, to monitor the stability of pulsars, the rotation of the Earth, and other periodic phenomena.
- In fundamental research, atomic clocks help to investigate physical constants and to clarify the question of whether they really remain invariant over time. Einstein's theory of relativity is not only verifiable with the help of atomic clocks but also opens up unimagined possibilities for higher geodesy. After all, Einstein's theory implies that masses influence not only space but also time.
- Einstein's theory of relativity is not only verifiable with the help of atomic clocks but also opens up unimagined possibilities for higher geodesy. Since time passes more slowly in the vicinity of large masses, this is also the case the closer one is to the center of gravity of the Earth. Consequently, a network of linked clocks with appropriate accuracy could make it possible to comprehensively measure the Earth's gravitational field. By observing the frequency shift of meshed optical clocks, it would be possible to tell whether, for example, the Earth's plates are shifting against each other or whether there is some form of geological activity at the location in question. The central reference parameter is the so-called geoid, which describes the hypothetical surface on which exactly the same gravitational force prevails everywhere on the globe. This reference surface for height measurements is by no means a sphere due to different mass distributions and different density fluctuations. This is because every plate tectonic movement and every earthquake changes the mass distribution of the Earth. Precise knowledge of the uplift or subsidence of land masses over time helps geoscientists determine where earthquakes and volcanic eruptions are particularly likely.
- Atomic clocks are indispensable for phase synchronization of fast data networks (distributed wireless networks) since data is transmitted over long distances using optical fibers. Here, the transmitting and receiving clocks must be synchronized at both ends of the transmission links, otherwise, no clear assignment of the individual bits can take place.
- In the banking sector, or more precisely in the area of high-speed trading on securities exchanges, the precise synchronization of trading clocks is a prerequisite for regulators to distinguish the order of receipt and execution of trading requests. In this way, market abuse can be detected. This is because traders using advanced, powerful, and fast computer networks would otherwise be able to manipulate securities markets by changing the prices of the securities they trade.
Credits:
Many thanks to Dr. Stefan Weyers, Head of the "Unit of Time" working group @ PTB for his extraordinary support of this article.
Image Source:
atomic clocks CSF1 and CSF2 & optical strontium clock: By courtesy of Physikalisch-Technische Bundesanstalt, www.ptb.de, both pictures are cropped and slightly distorted.
TWSTFT: Sattelite of our composing from Adobe Stock, Aleksandr Papichev
Author: Uwe Thomaschky
Remark:
At this point, we would like to give visitors to our website who are interested in the applications of our products a little insight. We ourselves are primarily concerned with the data acquisition requirements of our customers and are not geodetic scientists or involved in high-speed trading, nor do we build atomic clocks or satellite navigation systems. The following content is not to be understood as a scientific treatise but is a simplified presentation of these applications and might also reflect subjective impressions. This article was supported by the Time and Frequency Department @ PTB.